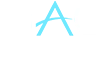

פרופ' עודד כאנר עבר את לימודיו לתואר ראשון באוניברסיטה העברית, וגם את לימודי המוסמך והדוקטורט במחלקה זואולוגיה באוניברסיטה העברית. תחומי המחקר בלימודים אלה עסקו בהתפתחות העוברית המוקדמת של עובר העוף.
פרופ' כאנר המשיך ללימודי פוסט-דוקטורט באוניברסיטת Berkeley בקליפורניה ארה"ב, במחלקה לביולוגיה מולקולרית והתפתחותית. שם הוא חקר את מנגנוני הדיפרנציאציה והמורפוגנזה הראשוניים בעובר קיפוד הים.
עם שובו ארצה השתלב פרופ' כאנר בסגל ההוראה של המכון למדעי החיים, במחלקה לביולוגיה של התא ושל בעלי חיים באוניברסיטה העברית. מחקרו כלל התמקדות ברקמות ייחודיות המעוררות את התפתחות ציר עובר העוף, הן ברמה המורפולוגית והן ברמה המולקולרית. כמו כן הרחיב פרופ' כאנר את תחום התמחותו לנושאי האבולוציה במחקר על גודל הגנום בדו-חיים.
פרופ' כאנר יצא לשנת שבתון במחלקה לגנטיקה באוניברסיטת Stanford בקליפורניה, ארה"ב והשתלם שם בתחום הביו-אינפורמטיקה והאבולוציה. עם חזרתו ארצה הוא התמנה לראש החוג למדעי המעבדה הרפואית במכללה האקדמית הדסה ושרת בתפקיד זה 17 שנים.
כיום משמש פרופ' כאנר כראש בית הספר לבריאות, חברה ומדע ובמקביל הוא אמור להתמנות לראש החוג לתואר שני במדעי המעבדה הרפואית.
יצירת קשר
טלפון: 02-6291904;
דוא"ל: odedk@hac.ac.il
List of publications:
Khaner, O., Mitrani, E., and Eyal-Giladi, H. (1985). [20]*
Developmental potencies of the area opaca and the marginal zone areas of the early chick blastoderms.
J. Embryol. Exp. Morphol.89 235-241.
Khaner, O., and Eyal-Giladi, H. (1986). [34]
The embryo forming potency of the posterior marginal zone in stage X through XII of the chick.
Dev Biol.115 275-281.
Khaner, O., and Eyal-Giladi, H. (1989). [61]
The chick's marginal zone and primitive streak formation. I. Coordinative effect of induction and inhibition.
Dev Biol.134 206-214.
Eyal-Giladi, H., and Khaner, O. (1989). [41]
The chick's marginal zone and primitive streak formation. II. Quantification of marginal zone's potencies-temporal and spatial aspects.
Dev Biol. 134 215-221.
Khaner, O., and Wilt, F. (1990). [37]
The influence of cell interactions and tissue mass on the differentiation of the sea urchin mesomeres.
Development109 625-634.
Khaner, O., and Wilt, F. (1991). [53]
Interactions of different vegetal cells with the mesomeres during early stages of sea urchin development.
Development112 881-890.
Khaner, O. (1993). [7]
The potency of the first two cleavage cells in echinoderm development - the experiments of Driesch revisited.
Roux's Arch. Dev Biol. 202 93-97.
Okasaka, K., Frudakis, T., Killian, C., George, N., Yamasu, K.,
Khaner, O., and Wilt, F. (1994). [20]
Genomic organization of a gene encoding the spicule matrix protein SM-30 in the sea urchin, Strongylocentrotus purpuratus.
J Biol Chem. 269 20592-20598.
· The number in square brackets represents the number of citations of the paper in the literature (taken from Medline)
Wilt, F., Livingston, B., and Khaner, O. (1995). [11]
Cell interactions during early sea urchin development.
American Zool. 35 353-357.
Khaner, O. (1995). [26]
The rotated hypoblast of the chick embryo does not initiate an ectopic axis in the epiblast.
Proc Nat. Acad Sci USA. 92 10733-10737.
Khaner, O. (1996). [9]
Axis formation in half blastoderm of the chick: Stage at separation and the relative positions of fused halves influence axis development.
Roux's Arch. Dev Biol.205 364-370.
Zahavi, N., Reich, V., and Khaner, O. (1998). [4]
High proliferation rate characterizes the site of axis formation in the avian blastula-stage embryo.
Int J Dev Biol. 42 95-98.
Khaner, O. (1998). [11]
The ability to initiate an axis in the avian blastula is concentrated mainly at a posterior site.
Dev Biol. 194 257-266.
Levy, V., and Khaner, O. (1998). [5]
Left-right cell migration across the midline of the gastrulating avian embryo.
Dev Genet. 23 175-184.
Chipman, A., Haas, A., and Khaner, O. (1999). [8]
Variations in anura embryogenesis: yolk rich embryos of the Hyperoliidae.
Evol dev. 1 49-62.
Chipman, A., Haas, A., Tchernov, E., and Khaner, O. (2000). [14]
Variation in anuran embryogenesis: Differences in sequences and timing of early developmental events.
J Exp Zool. 288 352-365.
Chipman, A., Khaner, O., Haas, A., and Tchernov, E. (2001). [7]
Evolution of Genome size: what can be learned from anuran development.
J Exp Zool. 291 365-374.
Abu-Rumia, R., Khaner, O., and Sidow, A. (2003).
Functional evolution in the ancestral linage of vertebrate or when genomic complexity was wagging in morphological tail.
J Struct Funct Genomics. 3 45-52.
Khaner, O. (2007a).
The importance of the posterior midline region for axis initiation at early stages of avian development.
Int J Dev Biol. 51 131-137.
Khaner, O. (2007d).
Embryonic polarity and axis determination in the early avian blastoderm.Trends in Dev Biol.2 61-69.
Khaner, O. (2017).
Hefzibah Eyal-Giladi (1925-2017): over fifty years of embryological research in Israel
Int J Dev Biol. 61 121-126.
Reviews:
Khaner, O. (1993). [28]
Axis determination in the avian embryo.
Curr Top Dev Biol.Vol. 12 155-180.
Khaner, O. (2007b).
Vertebrate innovations during evolution.
Integrative Zoology2 60-67.
Khaner, O. (2007c).
Morphogenetic mechanisms for axis formation in the avian embryo:
a morphological approach.
Trends in Dev Biol.2 35-44.
Chapters in books:
Wilt, F., George, N., and Khaner, O. (1991).
Sea urchin micromeres, mesenchyme, and morphogenesis.
From: Gastrulation-Movements, Patterns, and Molecules. pp. 281-288.
Edit by Keller, R., & Clark, W. H., & Griffin, F. Plenum press, New York.
Research Interests
My research interests during the recent years were focused on the understanding of the morphology and evolutionary mechanisms that shape the vertebrate embryo. One path of my research is to study the crucial phases of axis determination and the establishment of embryonic patterns of the vertebrate embryos. The other path of my research is to study crucial evolutionary and developmental phases of the vertebrates.
Identification of an axis initiation center in the avian blastoderm:
The experiments I had carried out in recent years, emphasize the developmental abilities of an axis initiation center in the avian blastoderm. This center includes the posterior marginal zone, Koller's sickle, and the posterior epiblast layer. Its ability to initiate the embryonic axis resides mainly at stage X in the posterior side of a narrow midline region of the marginal zone and Koller's sickle. At stages XI-XII this ability is exhibited at the region which is located more anterior and lateral to the posterior marginal zone, and at stage XIII it concentrate mostly at the posterior midline region of the epiblast, but not of the hypoblast. The cells which are located in this region have organizer properties, and express different molecular factors that act during the process of axis initiation and mesoderm induction.This axis initiation center has developmental abilities which allow it to initiate a single embryonic axis and at the same time to prevent other regions that have also such abilities (Khaner, 2007a), (Khaner, 2007c), (Khaner, 2007d).
Mesoderm induction in the early avian embryo
In the avian embryo two unique layers of cells, the epiblast and the hypoblast, constitute the blastoderm before the primitive streak develops. It was suggested that the hypoblast induces competent cells in the epiblast to form the mesoderm.
Results of my work have suggested, however, that the hypoblast does not act by inducing the epiblast to form a primitive streak (Khaner, 1995). These results shed new light over the issues of mesoderm induction in the avian embryo.
I have also tried to determine the relative contribution of particular sub-regions of the chick's blastula to primitive streak initiation and axis formation. The results obtained demonstrated that there is a considerable autonomy of the epiblast layer and of the posterior marginal zone, to form a primitive streak and the embryonic axis (Khaner, 1996), (Khaner, 1998).
Localized zones of high cell proliferation have been thought to be important in determining several phases of axis formation at early stages of chick development. We followed the pattern of cell proliferation in the early avian blastoderms, and found that at stage XIII blastoderm, a relatively high labeling of cells was detected all around the regions of the posterior marginal zone, Koller's sickle and the posterior part of the epiblast. This may be associated with the major developmental ability of this particular region to initiate the embryonic axis (Zahavi et al., 1998).
The development of bilateral symmetry in the avian embryo as a model for the vertebrates
One of the aims of my research concerned the morphological mechanisms underlying the determination of bilateral asymmetry in the avian embryo. The morphogenetic contribution of the right and left halves of avian blastoderms during primitive streak formation was examined. The right or the left half, were stained with the fluorescent dye Rodamine Dextran Lysine (RDL). The two halves of the blastoderm were reunited. During development, only that half of the primitive streak which derived from the stained half blastoderm was marked, although in some cases few stained cells could be seen in the counterpart primitive streak. The prospective AP midline in these experimental blastoderms served as a functional border line between the two halves. The conclusion from these experiments was that the midline contains special properties which serve as a border and as a physiological barrier for the cells during gastrulation(Levy and Khaner, 1998).
The evolution of differences in genome size and their effect onembryonic development in amphibians
The existence of large inter-specific differences in genome size (C-value), without any noticed correlation with organismic complexity, has been dubbed the C-value paradox. Substantial differences in genome size are most prominent in amphibians, with values of about 2-20 pg/N (picograms DNA per cell) in anurans, and 30-160 pg/N in urodeles. The approach used by us was based on comparative development of several different species of amphibians. The development rate and pattern for each species was noted at different temperatures, and several parameters were measured at different stages. In addition, the expression time of key embryonic genes was determined. After collecting data for several species we compared all these parameters and correlated them to genome size (and other relevant factors) in order to find which processes in the embryonic development were affected by genome size, and in what way. (Chipman et al., 1999), (Chipman et al., 2000), (Chipman et al., 2001).
A complete analysis of the morphology, molecular and evolutionary mechanisms that shape vertebrate embryonic development
The evolutionary research was aimed at a comprehensive solution of homology relationships within vertebrate developmental cells, tissues and organ systems, as well as their regulatory gene families. We have quantified evolutionary innovation and examined some of the crucial evolutionary phases in regards to chordata and vertebrata development. Using the MacClade software, we could plot the evolutionary trees for each particular organ system, and the evolutionary tree that summarizes all of the organs evolutionary trees. Although morphological innovations of vertebrata took place in nearly every evolutionary phase, we identified four most crucial phases where significant evolutionary development of vertebrates occurred (Aburomia et al., 2003), (Khaner, 2007b).